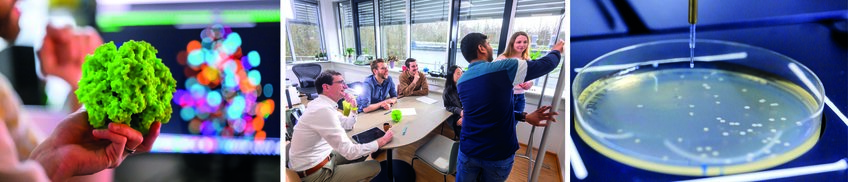
Cellular Operating Systems
Research in our group is organized around five central themes: (1) the biochemistry and evolution of natural CO2-fixing enzymes, (2) the design and engineering of new-to-nature CO2- and C1-converting enzymes, (3) the realization and optimization of in vitro synthetic metabolic networks, and their (4) transplantation into natural cells, including phototrophic chassis and (5) artificial cells. These efforts involve the development of novel genetic engineering tools & lab automation methods, as well as new in vitro biology-based and high-throughput technologies.
Research in all these areas is carried out in sub-teams of five to ten scientists. The different teams work in a highly inter- and transdisciplinary fashion and meet in regular subgroup meetings, which makes our research strongly team-oriented. Together we aim at transforming biology from an analytical-descriptive approach into a synthetic-constructive discipline that will allow us to find new solution for human needs (e.g., increased photosynthesis productivity or designer routes for the direct conversion of carbon dioxide into chemical and pharmaceutical building blocks or biofuels).
Our group is connected through several joint funding and joint projects with other local, national and international research groups, and entertains several collaborations with industry (from start-ups, to SMEs and global corporations). Together with the Department for Theoretical and Computational Biophysics at the MPI for Multidisciplinary Sciences, our group engages in a long-term collaboration with a Max Planck Partner Group “Quantum and Molecular Dynamics of enzymatic CO2-fixation” of Dr. Esteban Vöhringer-Martinez in Chile
Learn more about:
Evolution and Biochemistry of natural CO2-fixing enzymes
Engineering of new-to-nature CO2- and C1-converting enzymes
in vitro synthetic metabolic networks
Transplantation of new CO2-metabolism into natural cells
Phototrophic chassis
Design and realization of artificial organelles and cells
Return back to:
Department Overview
Group Overview