Biomechanics of membrane reshaping
Mechanical forces are integral to many cellular processes, often responsible for remodeling of cellular membranes. Though these membrane-reshaping processes differ in their purpose (e.g. cell differentiation, division or motility; intracellular membrane trafficking), common biomechanical principles can be drawn for application of forces during them. To reveal these fundamental principles, we use well-established and amenable membrane remodeling system of clathrin-mediated endocytosis in yeast. We develop and apply advanced fluorescence microscopy tools (FRET techniques and biosensors etc.) and combine them with powerful yeast genetics and biochemistry to answer the following questions:
1) How much force is required for endocytic membrane reshaping? How do endocytic proteins transmit this force to bend the membrane? Which physical and endocytic factors are important for successful force transmission?
2) How is the endocytic protein machinery mechanically organized and how it evolved towards a robust and efficient force-generating machine?
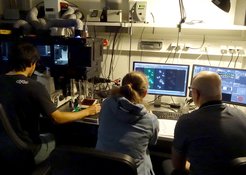
Mechanobiology of endocytic vesicle formation
During endocytosis, a small area of the plasma membrane reshapes into a closed vesicle. This requires considerable force provided by endocytic proteins and the polymerizing actin cytoskeleton. This force is needed to counteract membrane tension and (often huge) turgor pressure working against endocytosis (e.g. in yeast and other cell-walled organisms, turgor pressure reaches values similar to pressure inside a bike tyre).
To follow forces required for endocytic vesicle formation, we employ FRET-based tension sensors, the tiny molecular dynamo/Newton-meters able to measure piconewton forces directly in living cells (developed by Prof. C. Grashoff and colleagues). We implemented them into the indispensable protein linker Sla2-Ent1, which transmits force of the polymerizing actin for plasma membrane invagination (Skruzny et al., 2012). Using sensors of different sensitivity, we measured force needed for endocytic vesicle budding. We also analyze how endocytic force requirements change under various physical conditions or in cells with altered endocytic machinery.
In collaboration with M. Garcia-Alai team (EMBL Hamburg) we also continue to functionally and structurally characterize the Sla2-Ent1 protein linker, the critical force transducer of endocytosis (Skruzny, Desfosses et al., 2015; Garcia-Alai, Heidemann et al., 2018).
The functional architecture of the endocytic protein machinery
For understanding mechanobiology of endocytic membrane remodeling, the knowledge of its protein machinery is of utmost importance. This is however not an easy task given that dozens of endocytic proteins assemble at diffraction-limited spot of the endocytic site. We employ FRET, a microscopy method able to detect nanometer distances between fluorescently-labeled proteins, to map the nanoscale architecture of endocytic proteins and their rearrangements at the endocytic site. We recently comprehensively mapped the protein organization of the endocytic coat, a central multiprotein structure of the endocytic machinery (Skruzny et al., 2020). Now we analyze the other ever important endocytic protein assembly: WASp/Myo complex, responsible for the activation and regulation of force-generating actin cytoskeleton during endocytosis.
In addition, we extend our FRET analyses to other membrane-remodeling protein complexes and continue to implement new fluorescent proteins and FRET tools to our studies (reviewed in Skruzny et al., 2019).
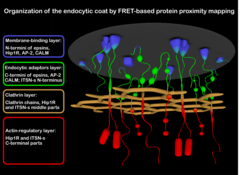